Radio Frequency High Pressure Plasmas
High-pressure, inductively coupled plasmas (ICP) have been used for variety of scientific and industrial applications. In such a plasma source, a helical coil is used to couple radio frequency (RF) power to the plasma using a capacitive impedance matching network. These plasmas are characterized by the presence of high concentration of reactive species and high enthalpy. Such plasmas find applications over large gas pressure range from a few Torr to atmospheric pressures. The applications of these plasmas require high-density (~ 1011-13 cm-3), uniform plasmas over large volumes (~1000-2000 cc). These high-pressure plasmas have applications in reducing drag on the supersonic vehicles, reducing radar cross sections by instantly forming a microwave absorbing or reflecting medium about an aircraft, their use as an agile mirror for scanning radar, as a source of atomic excitations (provides large number of reactive species), materials processing, exhaust gas processing, and biological decontamination.
One of the major issues associated with such high-pressure discharge is the high power budget required to initiate and sustain these discharges. Since ionization efficiency is sensitive to the electron distribution function, the reduction of higher energy electrons at higher gas pressure results in a lower production rate of electron-ion pairs. In addition two-body and three-body recombination become the significant loss mechanisms.
Goal
Create and Sustain high-density, large-volume high pressure plasmas of air constituents such as nitrogen, oxygen and air with moderate to low power budget.
We are investigating the following research topics to reduce the power requirement to create and sustain the plasma discharge.
- Design of an efficient inductive RF coupling system to the plasma by improving the antenna and the capacitive matching system. The excitation of suitable modes that will lead to better wave penetration both radially and axially, increase in the plasma resistive load and minimize resistive losses in the match and antenna feed system.
- A suitable of mix of monoatomic noble gases (Ar, Xe) that have lower recombination coefficients compared to the diatomic gases, reduced excitation of rotational/vibrational states and do not form negative ions.
- Once gas breakdown has occurred and the plasma discharge is initiated, the rf power can be much more efficiently absorbed by the plasma through inductive coupling of the wave and sustain the discharge at lower power.
- We hypothesized that if we could ionize a seed gas (2-8 mTorr of tetrakis (dimethylamino) ethylene or TMAE) by (193 nm) ultraviolet laser photon absorption, then we could efficiently couple radio frequency power to the plasma at higher gas pressures and sustain the plasma at much lower radio frequency powers.
Experimental Features:
- Excimer Laser (Lumonics Pulsemaster PM-842):
- 193 nm (uv) beam of output cross section 2.8x1.4 sq. cm
- (1/2 max) pulse width 20(±2) ns.
- Maximum Laser energy 300 mJ.
- Two radio frequency generators at 13.56 MHz are used to couple power to the antenna using an efficient capacitive impedance matching network.
- A 10 kW solid-state unit (CX10K-S, Comdel, Inc.) with variable duty cycle (90%-10%) and variable pulse repetition frequency (100 Hz-1 kHz) and very fast (microsecond) turn-on/off time.
- Another one is a 25 kW solid-state/triode unit (CXH25K, Comdel, Inc.) with variable duty cycle and large (5 kW) fold back power.
- A Five turn helical antenna is used, in conjunction with a capacitive matching network, to inductively couple rf power to the plasma.
- The two plasma chambers are Pyrex glass tubes of inner dimensions 5 cm x 150 cm and 10 cm x 150 cm.
- A turbo-molecular pump is used to pump the plasma chamber down to a base pressure of 3-5 x 10-6 Torr.
- Flow controllers and mixers are used to control the gas concentrations.
- A high-speed swirl gas injection system has been used to improve the gas flow system. In addition to an axial gas flow, four diagonally opposite holes, on 8 cm diameter circle, tangentially inject the gas into the chamber. Swirl flow keeps the plasma off the inside walls of the chamber by creating a gas layer. This arrangement improves the thermal conductivity, reduces the excess heating of the plasma chamber and permits longer experimental runs without damaging the Pyrex chamber.
- LabView programs have been written for automated system control and data acquisition.
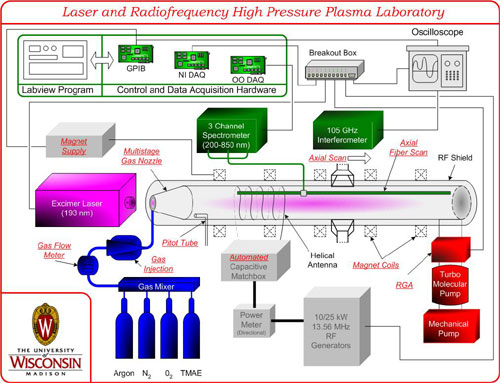
Diagnostics:
- Millimeter Wave Interferometry: A 105 GHz millimeter wave interferometery at high gas pressures to determine line average plasma density, the effective collision frequency and plasma decay parameters.
- At such high gas pressures, the mm wave propagating through the plasma arm undergoes phase change as well as strong attenuation. The wave amplitude attenuation is caused by the presence of high collisionality. In this situation the plasma density has a complex dependence on phase change as well as amplitude change and therefore, the plasma density estimate using only phase change data is inaccurate.
-
We use a measurement and analysis technique where both amplitude change and phase change data are used simultaneously to uniquely determine both plasma density and effective collision frequency. The treatment does not limit the application of interferometry to the relative values of collision frequency and hence can be used for measurements both at low and high gas pressures.
Figure 2: Interferometer trace in argon showing a nearly cut-off density ~ 9 x 1013 cm-3 using five turn helical antenna. - Spectroscopic Measurements: A three channel Ocean Optics Spectrometer is used to record the plasma spectral emission perpendicular to the plasma column axis. Each channel is a single grating spectrometer (1200 line/mm, optical resolution ~ 0.3 nm FWHM), which counts photons using a linear CCD array (2048 pixels). Samples are taken over the following ranges: 200-500 nm, 400-700 nm, and 600-850 nm.
- The primary spectroscopic measurements that have been performed are the atomic excitation temperature Tex and the molecular vibrational temperature Tvib.
- Residual Gas Analyzer: In the gas mix plasma, it is crucial to determine the chemical composition of the gas. It helps determine an optimal noble gas mix needed to bring down the RF power required to initiate and sustain the discharge.
- Antenna Wave Impedance: Antenna wave impedance measurements by network analyzer, using conjugate match principle, have been used to obtain Antenna-II code predicted plasma density.
Research Progress Highlights:
- Laser created and accurately measured high density (1013/cm3), large volume (500 cm3) low temperature (Te=0.3 eV) plasmas as a seed for nitrogen at up to 760 Torr. [1]
- First observation and publication of super excited, laser-produced seed gas states which cause delayed ionization and increased lifetime in nitrogen at up to 760 Torr at 1012-13/cm3 densities. [1]
- Obtained and published results of long pulse (4 ms), large volume (500 cc) 2.2 kW radiofrequency sustainment of laser-initiated seed plasmas (5x1012/cm3) at 150 Torr pressures which is twice that of radiofrequency creation alone [2].
-
We have recently further improved the experimental facility to obtain a large volume plasma (2500 cc) of plasma density ~ 1×1012 - 9×1012 cc in the pressure range 100 - 700 Torr at 2 kW RF power[3, ICOPS 02].
Figure 3: Comparison of laser initiated-rf sustained and rf initiated-rf sustained argon plasma density at modest rf power of 2.0 kW. Figure 4: Laser initiated-rf sustained argon plasma at 600 Torr. Plasma volume in 10 cm diameter tube is ~ 2500 cc. Note density is measured 10 cm away from the source region. -
Obtained high density (~ 9 x 1013 - 5 x 1012/cc), large volume plasmas at 10-250 Torr with just 0.5 W/cm3 power densities of argon and mixed nitrogen/argon using a new matching and antenna feed system with radiofrequency alone [4, ICOPS 02].
Figure 5: RF produced air plasma at 100 Torr with gas flow. -
We have investigated and confirmed that gas flow improves the plasma density and volume by an order of magnitude. The strong axial temperature gradient present during static discharge causes the plasma to be contracted under the antenna. We have seen that gas flow substantially reduces the axial temperature gradient and produces an extended plasma volume (5000-6000 cc) of high density (~ 1013 cc) and lower effective collision frequency (~1010/s) at 700 Torr.
Figure 6: Axial plasma density profile for different gas pressure and different flow rates. Figure 7: Axial excitation temperature profile for 50 Torr argon gas at different power but same flow rate.
Relevant Publications
- K. Akhtar, J. E. Scharer, S. Tysk, and C. M. Denning, “Characterization of laser produced tetrakis (dimethylamino) ethylene plasma in a high-pressure background gas,” IEEE Transactions on Plasma Sciences, April 2004.
- K. L. Kelly, J. E. Scharer, E. S. Paller, and G. Ding, “Laser Ionization and Radiofrequency Sustainment of High-Pressure Seeded Plasmas,” Jour. Of Applied Physics, July 2002.
- G. Ding, J. E. Scharer and K. Kelly, “Diagnostics and Analyses of Decay Processes in Laser Produced Tetrakis(dimehty-amino) Ethylene Plasma,” Physics of Plasmas 8(1), 334 (2001).
- E. S. Paller, J. E. Scharer, K. Akhtar, K. Kelly, G. Ding, “Radiofrequency Initiation and Radiofrequency Sustainment of Laser Initiated Seeded High Pressure Plasmas,” Conference Proceeding (2001).
- G. Ding, J. E. Scharer and K. Kelly, “Effects of rapidly decaying plasmas on Langmuir probe measurements,” Jour. Of Applied Physics 84, 1236 (1998).