Helicon Plasmas
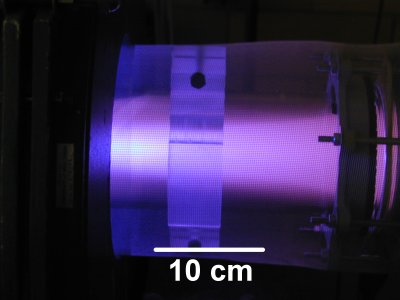
The helicon plasma facility was developed to investigate the physics and applications of these very efficient sources. Helicon plasmas are a class of radiofrequency-generated plasmas that are capable of very high densities, in the range of 1011 to 1014 cm-3. We have examined and continue to investigate helicon wave coupling, neutral depletion, double layer ion acceleration, and magnetic nozzle effects. Applications for helicons range from materials processing, to high-efficiency ion sources, to potential plasma thruster designs.
Facility
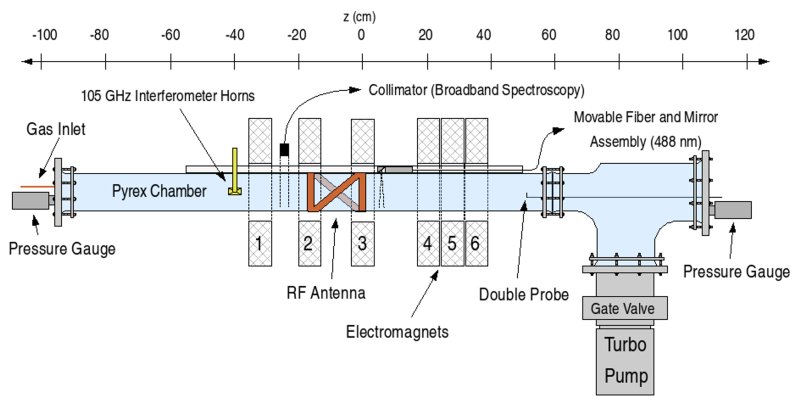
The helicon plasma lab consists of a Pyrex plasma chamber surrounded by a number of electromagnetic coils used to produce a DC magnetic field. The fill gas (typically argon) is fed into the upstream side of the chamber. The other end of the chamber has a mechanical and turbo pump attached for maintaining the vacuum. The plasma is created with 13.56 MHz RF that can be delivered as 6 ms pulses or in CW operation. The peak power available is 3 kW. A power meter is used to measure the forward and reflected power going into the plasma. The RF is matched to the half-turn double helix antenna with a variable capacitor matching network. Antenna source region magnetic fields up to 1.04 kG are possible with the source, with peak nozzle fields up to 1.5 kG.
Diagnostics
Our facility has a very wide range of diagnostics including:
- Tunable diode laser-induced fluorescence (LIF) (ion temperature and velocity)
- Broadband spectroscopy and collisional-radiative modeling (electron temperature and argon neutral density)
- 105 GHz microwave interferometry (electron density)
- Narrowband 488 nm spectroscopy (plasma density)
- Narrowband 443 nm spectroscopy (rf wave/optical emission correlation)
- Double Langmuir probe (plasma density, electron temperature)
- Wave magnetic field (B-dot) probe (helicon wave amplitude and phase)
- Diamagnetic loop (electron temperature perpendicular to magnetic field)
Laser-Induced Fluorescence (LIF): A tunable diode laser is used to excite an ion from a metastable state through a resonant transition. The ion then decays emitting a photon at a second wavelength. By tuning the diode laser, the ion energy distribution function along the laser path can be measured. Observing the Doppler shifting and broadening allows non-invasive measurement of the ion flow velocity as well as the temperature.
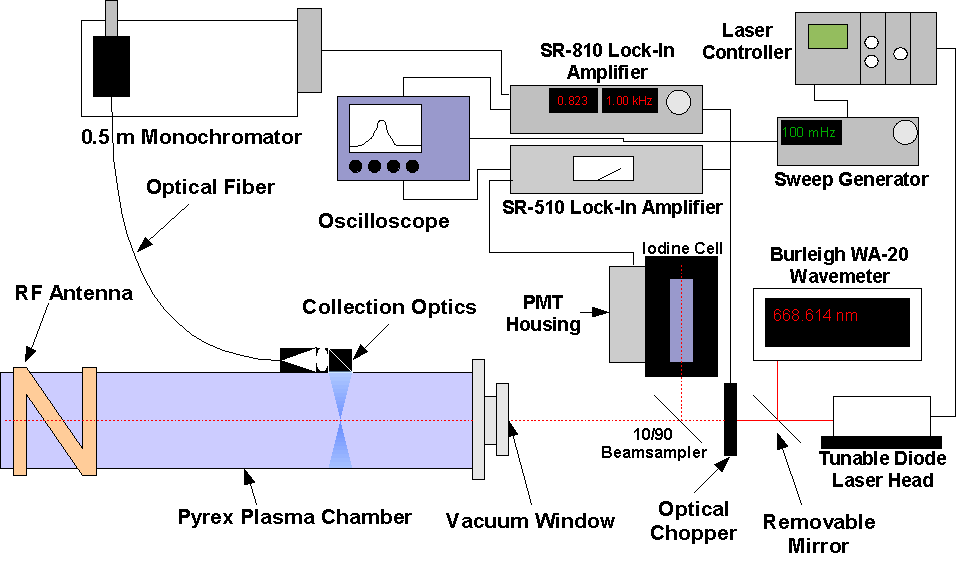
Broadband Spectroscopy with Collisional-Radiative Modeling: By observing argon ion (Ar II) and neutral (Ar I) lines, the densities of excited states can be determined. These densities can be compared with those predicted by computational codes that use collisional-radiative models. This technique can be used to non-invasively measure the electron temperature and the neutral density.
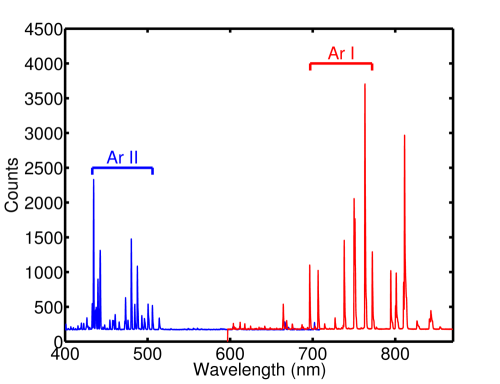
105 GHz Microwave Interferometry: The phase shift of microwaves that pass through the plasma is a linear function of the electron density. By comparing the phase of a wave that passes through the plasma with the phase of a wave that does not allows non-invasive measurement of this parameter.
Narrowband (488 nm) Spectroscopy: The optical emission intensity of a given ion line is proportional to the plasma density and the electron temperature, as well as the cross-section for excitation as a function of electron energy. Knowledge of the cross-section and the electron temperature can allow a measurement of the relative plasma density.
Narrowband (443 nm) Spectroscopy: By binning photons emitted at a given wavelength, the emission rates as a function of rf wave phase can be determined. This can be used to investigate rf wave/fast electron coupling.
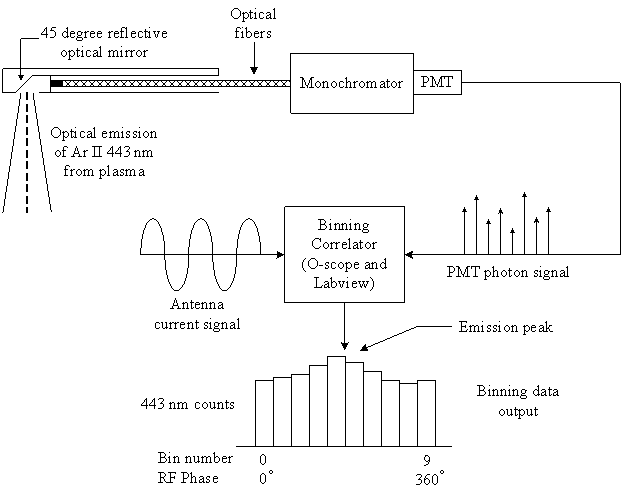
Magnetic Field Probe: The magnetic field probe is a 5 turn solenoid. Two wires from the solenoid are then formed into a twisted pair which lead to a hybrid combiner to reject common-mode signals resulting from electrostatic pickup. The probe measures the derivative of the magnetic field present within the solenoid. Measurements of the plasma wave amplitude and phase are possibe to characterize helicon wave coupling.
Double Langmuir Probe: The double Langmuir probe consists of two small sections of tungsten wire that are differentially biased that collect current from the plasma that is a function of the bias voltage. The resulting I-V curve can be used to measure the electron temperature and plasma density.
Diamagnetic Loop: A simple loop around the chamber can be used to measure the global time-varying magnetic field. This signal is proportianal to the component of the electron temperature that is perpendicular to the axially-directed magnetic field lines.
Relevant Publications
- Y. T. Sung, Y. Li, J. E. Scharer. "Fast, hot electron production and ion acceleration in a helicon inductive plasma," Physics of Plasmas, September 2016 [Copyright Notice]
- Y. T. Sung, Y. Li, J. E. Scharer. "Observation of warm, higher energy electrons transiting a double layer in a helicon plasma," Physics of Plasmas, March 2015 [Copyright Notice]
- M. Wiebold, Y. T. Sung, J. E. Scharer. "Ion acceleration in a helicon source due to the self-bias effect," Physics of Plasmas, May 2012 [Copyright Notice]
- M. Wiebold, Y. T. Sung, J. E. Scharer. "Experimental observation of ion beams in the Madison Helicon eXperiment," Physics of Plasmas, June 2011 [Copyright Notice]
- C. M. Denning, M. Wiebold, J. E. Scharer. "Observations of neutral depletion and plasma acceleration in a flowing high-power argon helicon plasma," Physics of Plasmas, July 2008 [Copyright Notice]
- S. M. Tysk, C. M. Denning, J. E. Scharer, and K. Akhtar, “Optical, wave measurements, and modeling of helicon plasmas for a wide range of magnetic fields,” Physics of Plasmas, March 2004.
- J. E. Scharer, A. Degeling, G. Borg, and R. Boswell, “Measurements of helicon wave propagation and Ar II emission,” Physics of Plasmas, September 2002.
- X. Guo, J. E. Scharer, Y. Mouzouris and L. Louis, “Helicon Experiments and Simulations in Nonuniform Magnetic Field,” Physics of Plasmas 6 (8), 3400 (1999).
- Y. Mouzouris and J. E. Scharer, “Wave Propagation and Absorption Simulations for Helicon Sources,” Physics of Plasmas 1(4), 875 (1998).